Nanomechanics Lab
In our lab, we pursue several applications of nanomechanical and electrical oscillators.
On the one hand, nanomechanical resonators are excellent sensors for classical and quantum measurements. They can be fabricated in many shapes and sizes, from cantilevers and doubly-clamped strings to extended membranes stretching over several millimeters. The resulting mechanical modes can be highly sensitive to magnetic, electrical or optical degrees of freedom. Our mission is to build novel instruments based on the most advanced nanomechanical devices, which we obtain through collaborations with leading nanofabrication groups.
On the other hand, nonlinear oscillators have many fascinating properties, both from a fundamental and an applied perspective. We are particularly interested in the physics of systems with a time-dependent potential, which is referred to as parametric modulation. When the modulation is strong enough and close to twice the resonance frequency, the system undergoes a spontaneous time-translation symmetry breaking and is locked into parametric oscillation states, which are promising as building blocks for analogue computation, topological pumping, and artificial neural networks. These experiments are done in very close collaboration with theorists.
One of our main goals is to develop experimental platforms to detect nuclear spin ensembles inside nanoscale objects with near-atomic spatial resolution. If successful, such magnetic resonance force microscopy (MRFM) can be useful for studying the structure and function of individual molecules and viruses. In our lab, we use silicon cantilevers to investigate how MRFM scans can be made faster and more precise. We find inspiration in clinical magnetic resonance imaging and nuclear magnetic resonance techniques, whose approaches we adapt to the special requirements of nanoscale, force-detected experiments.
Nils Prumbaum
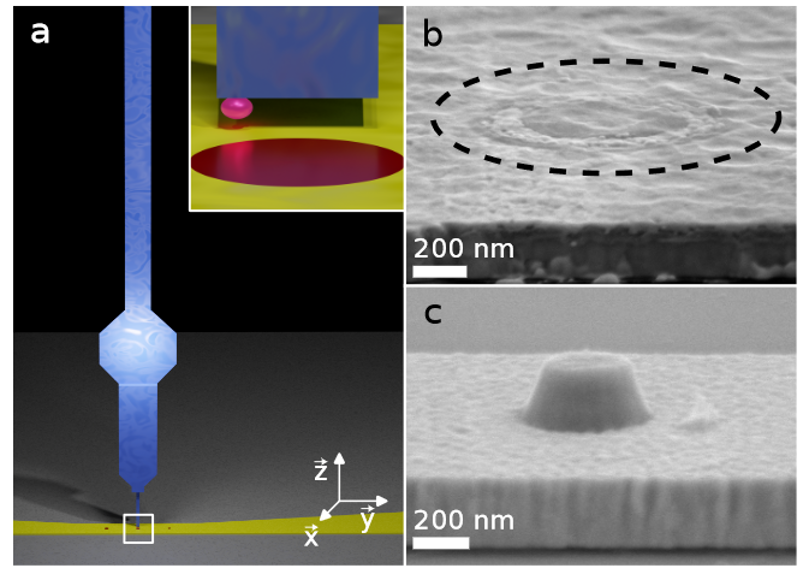
R. Pachlatko, N. Prumbaum, M. D. Krass, U. Grob, C. L. Degen, and A. Eichler, Nanoscale magnets embedded in a microstrip, Nano Letters 24, 2081 (2024)
M. D. Krass, N. Prumbaum, R. Pachlatko, U. Grob, H. Takahashi, Y. Yamauchi, C. L. Degen, and A. Eichler, Force-detected magnetic resonance imaging of influenza viruses in the overcoupled sensor regime, Phys. Rev. Applied 18, 034052 (2022)
U. Grob, M. D. Krass, M. Héritier, R. Pachlatko, J. Rhensius, J. Košata, B. A. Moores, H. Takahashi, A. Eichler, and C. L. Degen, Magnetic resonance force microscopy with a one-dimensional resolution of 0.9 nanometers, Nano Letters 19, 7935 (2019)
Silicon nitride membranes have become promising candidates for force sensing due to their exceptionally high-quality factors. We reported the first experimental demonstration of scanning force microscopy using a membrane resonator as force sensor in 2021. Since then, we have been striving to implement more functionality needed for MRFM, such as a high-finesse optical cavity, magnetic force sensing, spin inversion pulses, and low-temperature operation. In addition, we theoretically research various protocols for spin detection with membrane sensors.
Diego Visani, Shobhna Misra, Geena Benga, Vincent Dumont, collaboration with Albert Schliesser (NBI) and Javier del Pino (UKON)
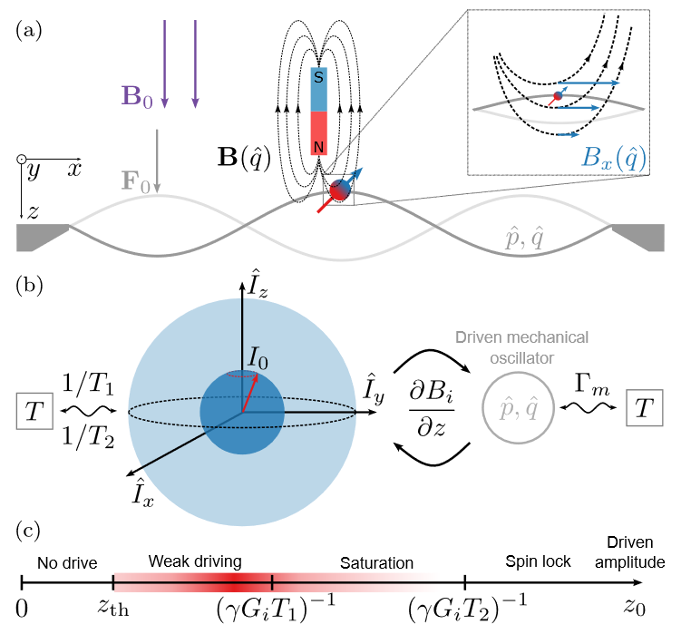
D. A. Visani, L. Catalini, C. L. Degen, A. Eichler, and J. del Pino, Near-resonant nuclear spin detection with high-frequency mechanical resonators, arXiv:2311.16273
A. Eichler, Ultra-high-Q nanomechanical resonators for force sensing, Materials for Quantum Technology 2, 043001 (2022)
D. Hälg, T. Gisler, E. C. Langman, S. Misra, O. Zilberberg, A. Schliesser, C. L. Degen, and A. Eichler, Strong parametric coupling between two ultra-coherent membrane modes, Phys. Rev. Lett. 128, 094301 (2022)
Silicon nitride string resonators typically have lower masses than membranes and are therefore even more sensitive to forces. We have started researching how such string resonators could be used as force sensors in a scanning force microscope. In a first step, we are analyzing the interplay between different mechanical string modes and the noise correlations.
Bhavesh Kharbanda, Luis Martins Mestre, collaboration with Tobias Kippenberg (EPFL) and Nils Engelsen (Chalmers)
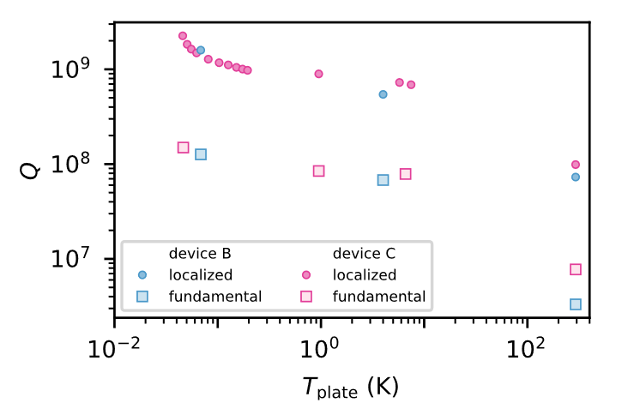
T. Gisler, M. Helal, D. Sabonis, U. Grob, M. Heritier, C. L. Degen, A. H. Ghadimi, and A. Eichler, Soft-clamped silicon nitride string resonators at millikelvin temperatures, Phys. Rev. Lett. 129, 104301 (2022)
Another goal we have is to convert nanomechanical resonators into mass sensors on the single-molecule level. If a resonator can identify various molecules based only on their specific masses, a new generation of rapid biological diagnostics tools will be within reach. In order to approach this goal, we try to understand how surface defects influence dissipation and frequency fluctuations, and how these noise sources can be suppressed.
Geena Benga, Bhavesh Kharbanda, Diego Visani, Vincent Dumont, Antonius Armanious, John Abendroth, collaboration with Silvan Schmid and Martina Marchetti-Deschmann (TUW) and Albert Schliesser (NBI)
A single nonlinear system with parametric modulation is an ideal testbed for investigating the interplay of driving, dissipation, and multiple stable states. Applying various drives and white noise, we engineer situations that are analogous to effective multi-well potentials, and explore statistical transitions between these wells. There is a great wealth of phenomena to be discovered in such systems, and it can be great fun. Our prime experimental resources are electro-micromechanical devices from Stanford.
Luis Martins Mestre, Vincent Dumont, collaboration with Oded Zilberberg and Javier del Pino (UKON) and Tom Kenny (Stanford)
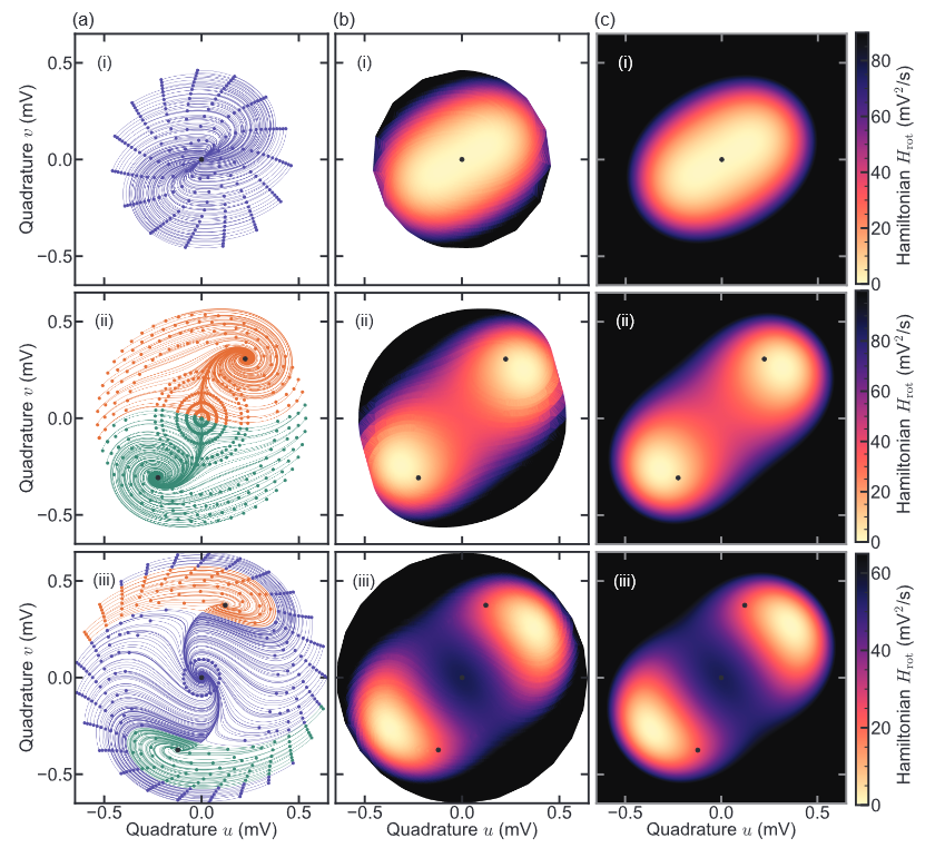
V. Dumont, M. Bestler, L. Catalini, G. Margiani, O. Zilberberg, and A. Eichler, Hamiltonian reconstruction via ringdown dynamics, under review, arXiv:2403.00102
G. Margiani, S. Guerrero, T. L. Heugel, C. Marty, R. Pachlatko, T. Gisler, G. D. Vukasin, H. K. Kwon, J. M. L. Miller, N. E. Bousse, T. W. Kenny, O. Zilberberg, D. Sabonis, and A. Eichler, Extracting the lifetime of a synthetic two-level system, Applied Physics Letters 121, 16 (2022)
When several parametric oscillators are coupled together, they form networks that can formally resemble coupled Ising spins. In tight experiment-theory synergy, we explore the conditions under which the resonator-spin analogy holds, and where (and how) it breaks down. Our current experiments are based on electrical or nanomechanical systems that offer strong coupling and/or low dissipation, and that allow us to explore various limits of network behavior.
Gabriel Margiani, Luis Martins Mestre, Vincent Dumont, collaboration with Oded Zilberberg (UKON)
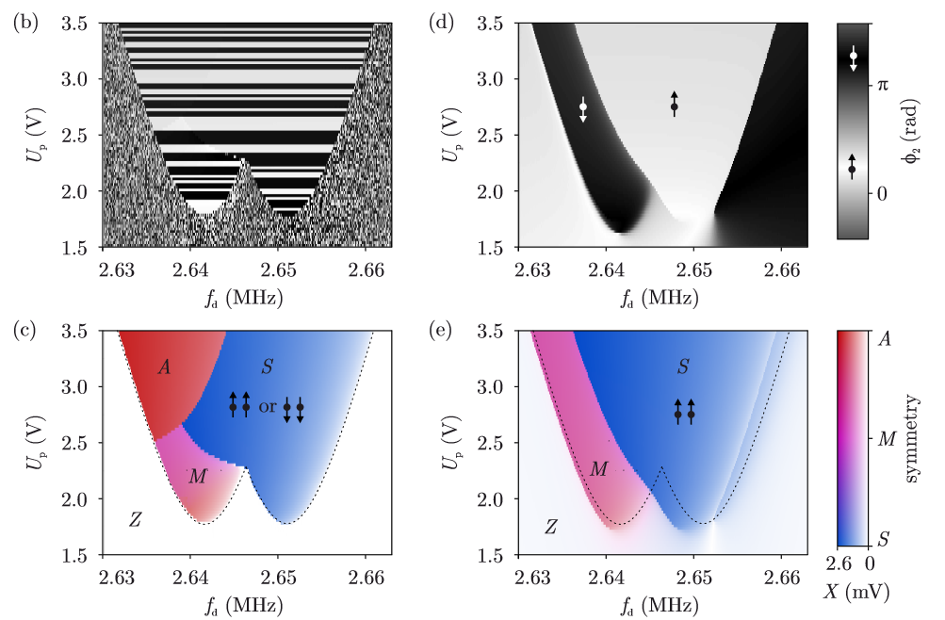
P. Álvarez, D. Pittilini, F. Miserocchi, S. Raamamurthy, G. Margiani, O. Ameye, J. del Pino, O. Zilberberg, and A. Eichler, A biased Ising model using two coupled Kerr parametric oscillators with external force, in press at Phys. Rev. Lett.
T. L. Heugel, R. Chitra, A. Eichler, and O. Zilberberg, Proliferation of unstable states and their impact on stochastic out-of-equilibrium dynamics, under review, arXiv:2307.13718
G. Margiani, J. del Pino, T. L. Heugel, N. E. Bousse, S. Guerrero, T. W. Kenny, O. Zilberberg, D. Sabonis, and A. Eichler, Deterministic and stochastic sampling of two coupled Kerr parametric oscillators, Phys. Rev. Research 5, L012029 (2023)